One of the properties of superconductors most easy to demonstrate, and also the most dazzling, is the Meissner Effect. Superconductors are strongly diamagnetic. That is to say that they will repel a magnet. Imagine a 'perfect' conductor of electricity that simply has no resistance to the flow of an electric current. If a conductor of electricity is moved into a magnetic field, Faraday's Law of Induction would lead us to expect an induced electrical current in the conductor and its associated magnetic field which would oppose the applied field. The induced electrical current would not dissipate in a `perfect' conductor, and thus the associated magnetic field would also continue to oppose the applied field. Conversely, if the `perfect' conductor was already in a magnetic field, and then that applied field was removed, the same physical law would indicate that an electrical current and its associated magnetic field would appear in the conductor which would attempt to oppose the removal of the applied field. If we were to do an experiment in which we placed a magnet on top of a material that by some process then became a `perfect' conductor, we would see no physical effect on the magnet. However, were we to attempt to remove the magnet, only then would we feel an opposing force.
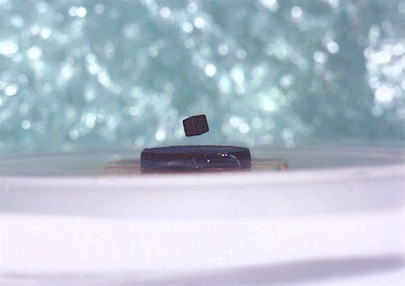
A superconductor is fundamentally different from our imaginary 'perfect' conductor. Contrary to popular belief, Faraday's Law of induction alone does not explain magnetic repulsion by a superconductor. At a temperature below its Critical Temperature, Tc, a superconductor will not allow any magnetic field to freely enter it. This is because microscopic magnetic dipoles are induced in the superconductor that oppose the applied field. This induced field then repels the source of the applied field, and will consequently repel the magnet associated with that field. This implies that if a magnet was placed on top of the superconductor when the superconductor was above its Critical Temperature, and then it was cooled down to below Tc, the superconductor would then exclude the magnetic field of the magnet. This can be seen quite clearly since magnet itself is repelled, and thus is levitated above the superconductor. For this experiment to be successful, the force of repulsion must exceed the magnet's weight. This is indeed the case for the powerful rare earth magnets supplied with our kits. One must keep in mind that this phenomena will occur only if the strength of the applied magnetic field does not exceed the value of the Critical Magnetic Field, Hc for that superconductor material. This magnetic repulsion phenomena is called the Meissner Effect and is named after the person who first discovered it in 1933. It remains today as the most unique and dramatic demonstration of the phenomena of superconductivity.
On account of the polycrystalline nature of a typical ceramic superconductor, the Meissner Effect appears to be a bulk phenomena. This can be demonstrated by stacking two or more superconductor disks. With the addition of each disk, the magnet will be levitated higher. This result is particularly advantageous if the Meissner Effect is being demonstrated to an audience with the help of an overhead projector.
Another interesting observation is that the levitated magnet does not slide off the superconductor. This seemingly stable equilibrium is actually a manifestation of Flux Pinning, a phenomena uniquely associated with Type II superconductors, of which our high temperature ceramic superconductors are examples. Here lines of magnetic flux associated with a magnet can penetrate the bulk of the superconductor in the form of magnetic flux tubes. These flux tubes are then pinned to imperfections or impurities in the crystalline matrix of the superconductor thereby pinning the magnet.
In other words, what is happening is that you are initially forcing the magnetic field to exist in these non superconducting regions, by "squeezing" it though the cracks between the superconducting crystals. These regions of the material are surrounded by superconducting material. Think of a gallon jug, filled with water, that has a small pin hole in the bottom. The jug is the superconductor, the water is the magnetic field. This superconducting material will not allow a magnetic field to pass though it, in much the same way the jug will not allow the water to pass though it. However, the tiny non superconducting regions will allow the magnetic field to pass though, in the same way the pin hole in the jug allows the water to pass though. When you lift the magnet up, the force of gravity acting on the pellet (F=ma first semester physics stuff), is not great enough to force the trapped magnetic field to pass though the superconducting material, hence, like a weight on a string, you can lift the pellet. The string in this case is the magnetic field, and the weight is the superconductor.
Meissner Effect Demonstration
The procedure below, will guide the experimenter through a demonstration of the Meissner Effect in a cookbook fashion, step by step.
The shallow dish-like depression in the styrofoam container for the kit, or a third of an inch high portion of the bottom of a styrofoam coffee cup, can be used for holding liquid nitrogen for the experiment. To project a sharp image of the Meissner Effect with an overhead projector, use a very small dish so that the levitated magnet is less than an inch from its glass plate.
Procedure
- ACTION
Carefully pour a small amount of liquid nitrogen into the dish or styrofoam cup until the liquid is about a quarter of an inch deep.
RESULT
The liquid boils furiously for a short while. Wait until it stops boiling. - ACTION
Using the provided tweezers, carefully place the black superconductor disk flat in the liquid until its top is just flush with the surface of the liquid nitrogen.
RESULT
Again, the nitrogen boils around the disk. Wait until this boiling stops too. - ACTION
Again using the tweezers, pick up the provided magnet, and attempt to balance it on top of the superconductor disk.
RESULT
Instead of settling down onto the surface of the superconductor, the magnet will simply `float' a few millimeters above the superconductor.
This is a demonstration of the Meissner Effect.
Precautions
- When pouring liquid nitrogen please be careful to prevent any splashing.
- Conduct the experiments in a well-ventilated room.
- Do not touch any items immersed in the liquid nitrogen with your hand until they have warmed to room temperature. Use the provided tweezers to add and remove items from the liquid nitrogen.
This experiment can also be conducted by placing the magnet on top of the superconductor before it is cooled in liquid nitrogen. As predicted by the Meissner Effect, the magnet will levitate when the temperature of the superconductor falls below its Critical Temperature. As explained earlier, there is no material other than a superconductor which could have shown this effect.
If you carefully set the magnet rotating, you will observe that the magnet continues to rotate for a long time. This is a crude demonstration of a frictionless magnetic bearing using the Meissner Effect. The rotational speed of a cube-shaped magnet can be increased by using a plastic drinking straw to blow a stream of air at one of the edges or corners of the cube. Another way to increase the magnet's rotational speed is to cut out a small rectangular hole in a piece of paper. The hole is positioned over the levitated magnet such that half of the magnet projects above the plane of the paper. A stream of air directed along the upper surface of the paper will cause the magnet to rotate rapidly.
The cubical magnet naturally is slowed by the resistance of air. Consequently, it can be expected to stop after a while. A cylindrical magnet will rotate for much longer since it is rotationally streamlined. However, the cubical magnet makes this demonstration much more graphic. A research group at Cornell University has demonstrated a frictionless superconducting bearing that can turn at a rate of one million rotations per minute. A bearing constructed in this manner, using the Meissner Effect is much more convenient and safe than a conventional magnet bearing because of the `self-centering' nature of the Meissner Effect on account of flux pinning.
All Kits from Colorado Superconductor Inc. are equipped to demonstrate the Meissner Effect. The Comparison Kits contain both a yttrium-based (YBa2Cu3O7) and a bismuth-based (Bi2Sr2Ca2Cu3O10) superconductor. Both superconductors exhibit the Meissner Effect, however, if the disks are carefully removed from the liquid nitrogen bath, while magnet is still levitated, the bismuth-based material will continue levitating the magnet for a considerably longer time than the yttrium-based superconductor. This is because the bismuth-based superconductor has a significantly higher Critical Temperature than to the yttrium-based one.
The Critical Temperature Kit and the Critical Temperature Comparison Kit both use the Meissner Effect to measure the Critical Temperature of superconductors.
Some Questions
- Why does the liquid nitrogen boil when you pour it into the dish? Why does it boil when you put the superconductor disk into it?
- When the nitrogen has evaporated, the magnet stays levitated for a short while longer. Why is this so? Can you think of any other experiments using this fact?
- If you push the levitated magnet with the tweezers so as to move it across the superconductor, it will resist movement. Why does this happen?
- How can you improve the operation of the model frictionless bearing in your Kit?
- There are many potential applications of the Meissner Effect, for example, magnetically levitated transport vehicles, frictionless bearings, low vibration mounts, etc. Can you think of other applications?
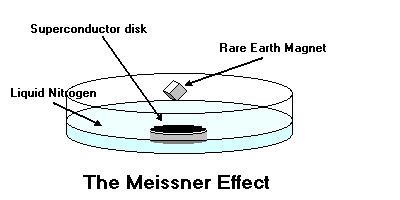
Information Courtesy of CSI Superconductors